Planets in and beyond our Solar System (Exoplanets)
Looking at planets away from Earth helps us better understand Earth, particularly their radiation budgets, dictated by how much radiation illuminates the planet throughout its orbit.
A Star’s Temperature and its Radiation Spectrum
Planets gain radiational energy from the stars they are orbiting. But not all stars emit the same type and intensity of radiation. The spectrum and amount of energy emitted depend on the star’s surface temperature (see the Radiation page). The total amount of radiation emitted is proportional to its surface temperature [K] to the fourth power or T4.
The peak wavelength of radiation emitted is inversely proportional to the surface temperature. The hotter the star, the shorter the peak wavelength (see the animation of a star’s thermal animation). The surface temperature of our Sun is 5700K, and a small but essential part of its light is in the deadly ultraviolet rays. Stars hotter than our Sun will emit even more UV, decreasing the chances that early, simple life forms can survive on a planet.
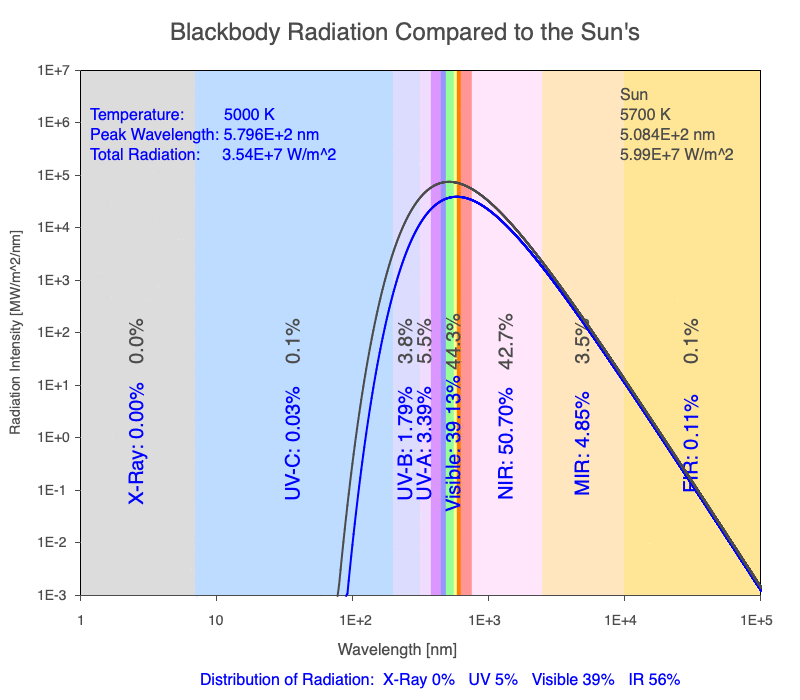
Diagram of the spectrum of radiation emitted from a star (blue line) based on its surface temperature. For comparison, the Sun’s spectrum is the black line.
Distance from the Star
Radiation emitted from a star travels radially outward. The energy moves away from the star in an expanding sphere. The surface area of a sphere is 4 π r², so the energy is being distributed over an ever-growing surface area that is proportional to the square of the distance traveled (r²). The energy/unit time/unit area (energy flux) decreases inversely to the rate of increase in the surface area of the sphere. Using I to represent the energy flux and r as the distance from the star, I(r):
I (at distance r from star) = I (at the star) / r²
or I(r) = I0 / r²
If we know the energy flux at one distance from a star, I(r1), as we do for the energy flux from the Sun reaching Earth, then we can calculate the energy flux at any other distance from the star, I(r2):
I(r1) = I0 / r12
I(r2) = I0 / r22
Rewriting each in terms of I0:
I0 = I(r1) * r12 = I(r2) * r22
Then: I(r2) / I(r1) = r12 / r22
Where r1 and r2 are two distances from the source.
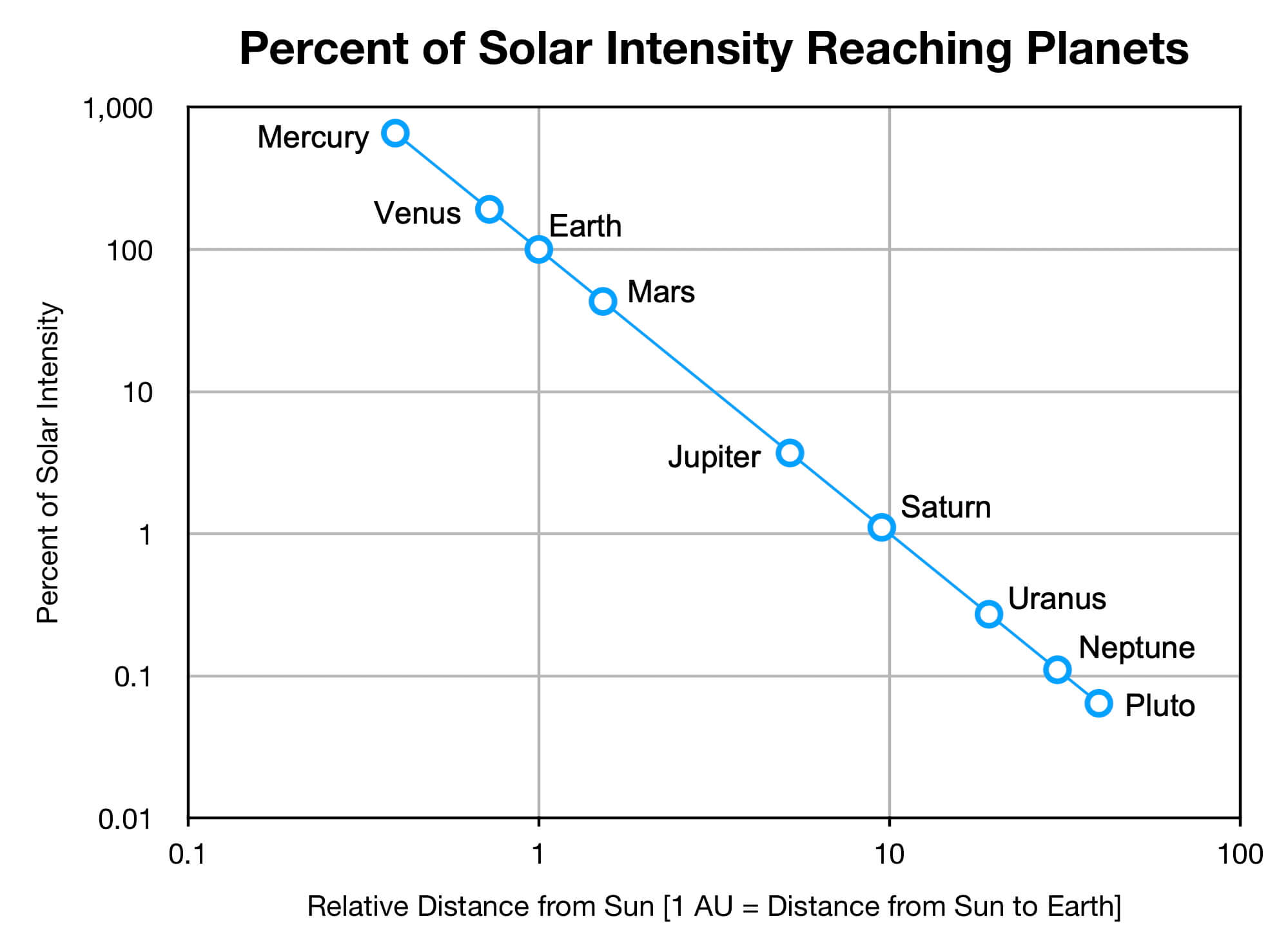
Based on the distance from the Sun, a graph of the percent solar radiation reaching a planet in our solar system compared to that reaching Earth.
Notice that Saturn is nearly ten times as far away from the Sun and it receives 1/10² or 1% of the energy compared to the sunlight reaching Earth.
Habitable Zone or Goldilocks Zone
The amount and type of radiation emitted from a star are two critical components that help create a climate that allows liquid water to exist on a planet. The Habitable or Goldilocks Zone defines the distances from the star where liquid water could exist. Cooler stars emit less radiation, and the Habitable Zone is closer to the sun. Conversely, hotter stars create a Habitable Zone further from the star.
The Habitable Zone is just a starting point. Earth Systems focuses on the interconnected processes needed to allow our planet to support and sustain life on it for most of its 4.5 billion years. Earth is in the habitable zone, but so are other planets in our solar system, yet only Earth has complex life. Why?
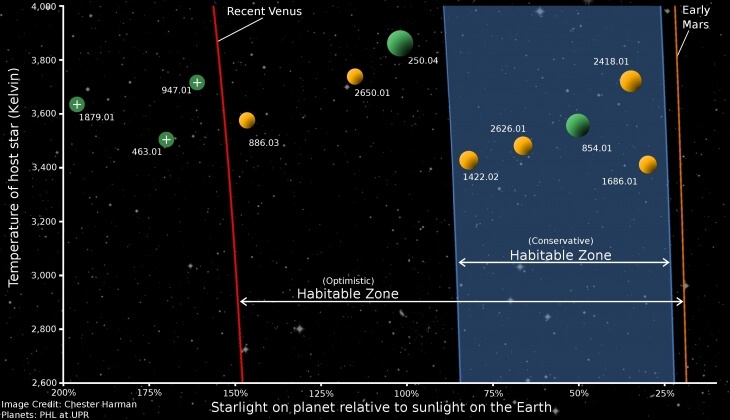
The above diagram shows optimistic and conservative habitable zone boundaries around cool, low mass stars. The numbers indicate the names of known Kepler planet candidates. Yellow color represents candidates with less than 1.4 times Earth-radius. Green color represents planet candidates between 1.4 and 2 Earth radius. Planets with “+” are not in the habitable zone. Image from Penn State.
Factoring in a Planet’s Orbital Parameters
Even if a planet were within the Habitable Zone, if the obliquity and/or eccentricy were too high, the dramatic seasonal temperature changes would challenge the survival of life. Annually going from deadly hot to frigidly cold would limit the chance of life thriving.
Although several planets in our solar system (see the table of orbital parameters), Mars has an eccentricity nearly six times that of Earth. Since humans have sent instrumentation to Mars, we have studied how the planet’s atmosphere responds to the significant changes in distance from the Sun. For example, surface air pressure changes by 25% as Mars moves from aphelion to perihelion. Why does Mars have such a large eccentricity? The considerable pull from its massive neighbor, Jupiter. This article studied the effects of the dramatic seasonal temperature change for highly eccentric orbits and predicted that enough water would remain liquid for life to form. Since changes in obliquity, eccentricity, and precession are caused by the gravitational pulls of the orbiting planets and Sun, each planet in experiences Milankovitch cycles. Earth’s cycles of relatively small perturbations in its orbital parameters have helped trigger the recent cycles of glacial periods. Other planets may have more substantial cyclical changes in their orbits, which could create long periods of harsh conditions, threatening the evolution of primitive life forms.
Orbital Parameters for the Solar System’s Planets
Planet | Obliquity¹ | Eccentricity |
Mercury | 0.1º | 0.2056 |
Venus | 177.4º | 0.007 |
Earth | 23.45º | 0.0167 |
Mars | 25.19º | 0.0934 |
Jupiter | 3.12º | 0.0484 |
Saturn | 26.73º | 0.0565 |
Uranus | 97.86º | 0.0473 |
Neptune | 29.56º | 0.0113 |
Pluto | 119.6º | 0.25 |
¹ Obliquities greater than 90º indicate that the planet is rotating from east to west rather than the Earth’s rotation from west to east.
Additional Factors to Consider
Although the star-planet connection is essential to consider when looking for exoplanets that support life, many other factors are critical to maintaining the planet’s climate that allows liquid water to exist. On Earth, liquid water has existed continuously once conditions cooled from the formation of the planet.
-
- Rate of planet rotation: faster-rotating planets have shorter days, higher average daily temperatures, and less diurnal temperature change than planets that rotate slower.
- The reflectance, specific heat, and density of the planet’s surface material affect the range of diurnal temperature change. A large range in daily temperatures creates greater stress on life to survive. Liquid water has one of the highest specific heat values of any naturally occurring molecule, and large bodies of water dramatically decrease the diurnal temperature change of our planet.
- An atmosphere is essential to keep liquid water on the planet (it would rapidly boil away into space without an atmosphere) and store more of the daytime heat that would offset a portion of the nighttime cooling.
- The presence of greenhouse gases, which absorb considerable amounts of the thermal infrared emitted from the warmed surface, increases solar radiation storage.
As scientists continue to scan the nearby galaxies for exoplanets that could support life, we will learn more about the essential energy flows and matter cycles required to support life.
Exoplanets: Planets Outside Our Solar System
In 1995, NASA’s Kepler Space Telescope detected the first known exoplanet. Since then, scientists and engineers have built and launched satellites dedicated to exploring the Universe for exoplanets. See the visualizations of the ones currently discovered here, and follow the stories of discoveries here. The variety has staggered the imaginations of even the experts. Here are a few that show the diversity of exoplanets.
One of the benefits of studying exoplanets is that it helps us think about Earth in new ways. Often the prevailing conditions are not questioned and explored thoroughly. Seeing worlds so different from ours helps us change our perspective of Earth, improving the questions that guide how we explore our complex world.
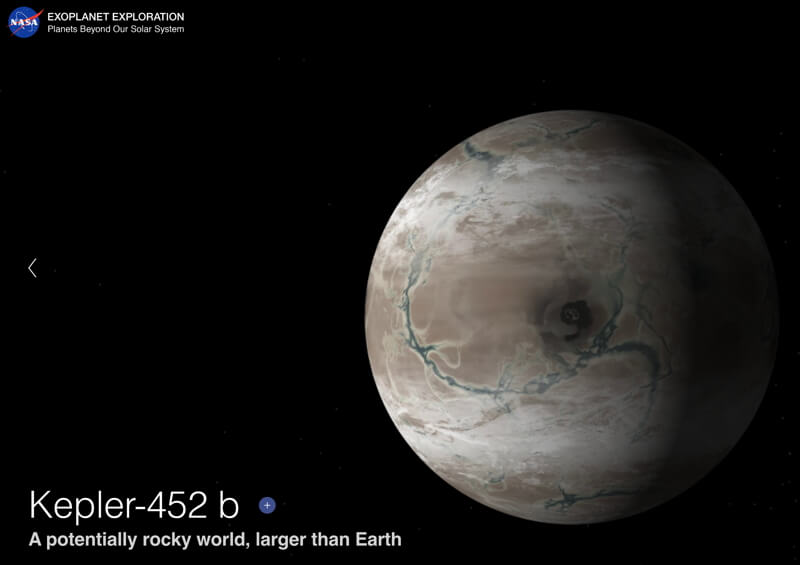
A hypothetical view of the Kepler-452b exoplanet from NASA.
Current Discoveries
Planets more hospitable to life than Earth may already have been discovered October, 2020
Big Ideas
- The first step in exploring an exoplanet is studying its energy budget, starting with the radiation emitted by its star.
- Next is to analyze the orbital parameters of the planet to analyze its climate stability.
- The types of surface materials and atmospheric gases determine how much solar radiation is absorbed and retained to minimize the planet’s climatic variability.
- Learning about worlds so different from ours helps us improve the questions that guide how we explore our complex planet Earth.
0 Comments