Earth’s Atmosphere
Overview
A planet’s atmosphere consists of the available atoms and molecules that are in the form of gases. Only those having kinetic energy below a given threshold are retained by the planet’s gravity. Which atoms and molecules will be part of the atmosphere depend on the planet’s surface and atmospheric temperatures, air pressure, and the chemistry of the atoms and molecules themselves.
Gases are the least dense form of a state of matter, so they are concentrated near the planet’s surface. They have an indefinite shape and volume and are relatively easy to move over the Earth’s surface. Moving air creates our weather and modifies climate regimes as it transports heat from the tropics toward the poles.
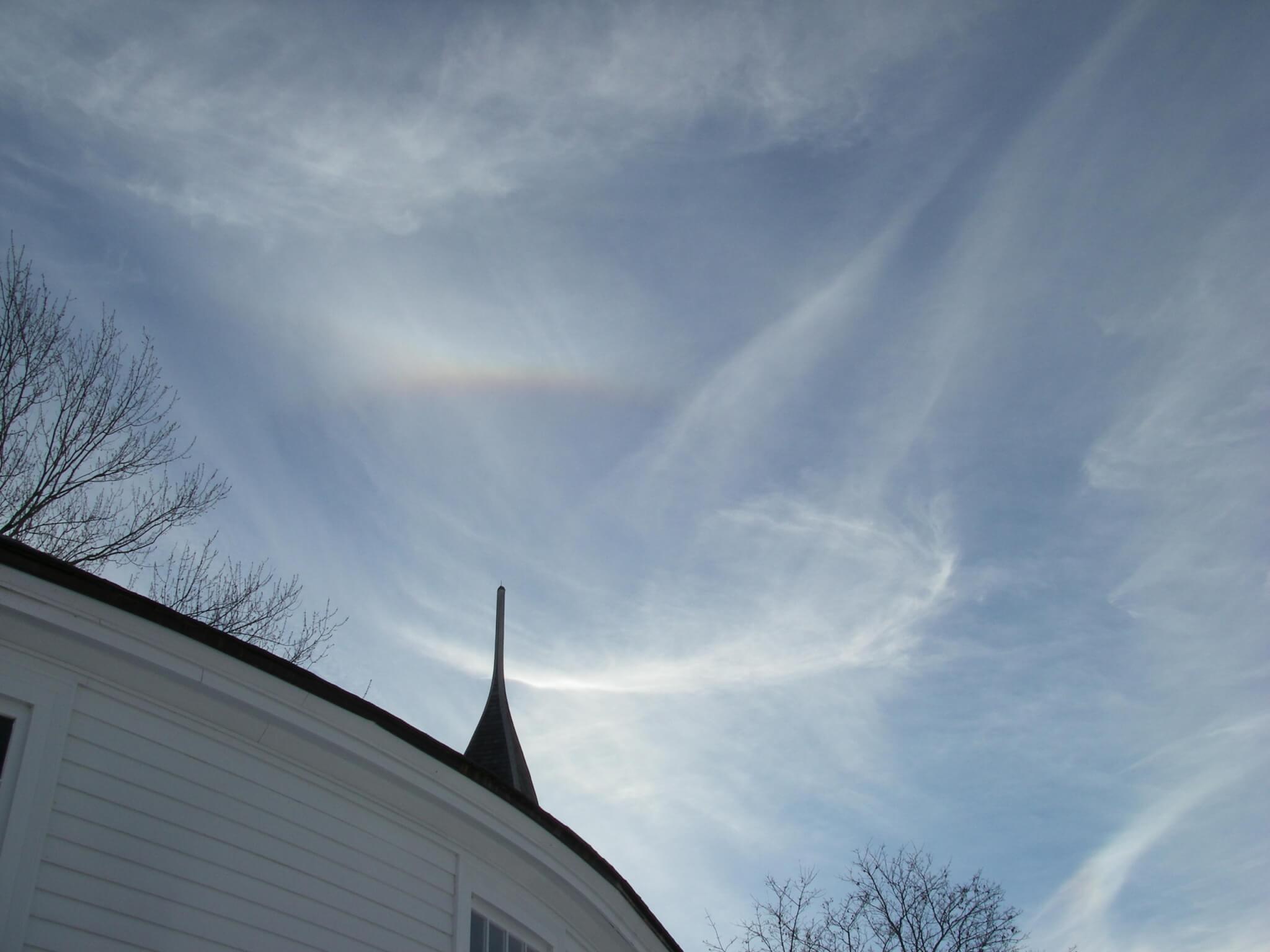
Gases are compressible, and air pressure is the weight of an air column extending vertically from the given location to the top of the atmosphere. Because air is compressible, the density of air increases toward the Earth’s surface. Roughly 75% of the mass of the atmosphere is within 10 km of the Earth’s surface. This is only 0.15% of the Earth’s radius, but this is enough to support life on Earth, create a complex energy budget by capturing and transporting large amounts of heat, drive the water cycle, and keep liquids available on our planet. Without an atmosphere, our oceans would have boiled away.
A Planet Without An Atmosphere
We don’t need to look far to study the effects of a body in space without an atmosphere – our moon! Because the moon’s gravity is not strong enough to maintain an atmosphere, its daytime surface temperatures reach 260ºF (127ºC) and nighttime temperatures plummet to -280ºF (-173ºC) – that is a 540ºF diurnal temperature change!
Evolution of Earth’s Atmosphere Over Time
Earth’s atmosphere has evolved over time due to a number of factors: cooling temperatures of our planet’s surface over time, gravity, temperature and solar activity of the Sun, the strength of Earth’s magnetic field over time, volcanic activity caused by plate tectonics, presence and abundance of photosynthesizing life, and technologically advanced life forms.
Major Gases in Earth’s Current Atmosphere
There are only four major gases found in Earth’s atmosphere, yet only three are well mixed throughout the lower layers of the atmosphere. Water is concentrated in the lowest layer of the atmosphere.
Chemical Composition of Earth’s Atmosphere
What’s in Our Milky Way?
Nature builds things with the available materials, so to understand what Earth had to work with when it formed, look at the chemical composition of the Milky Way:
-
- Hydrogen 73.97%
- Helium 24.02%
- Oxygen 1.04%
- Carbon 0.46%
- Neon 0.13%
- Iron 0.11%
- Nitrogen 0.10%
- Silicon 0.07%
- Magnesium 0.06%
- Sulfur 0.04%
Many of these atoms readily form gas, so they were either part of our early atmosphere or accumulated over time.
Nitrogen (78.1%) gas is in the form of two tightly bonded nitrogens, which is nearly inert to chemical reactions in this form. Since gaseous nitrogen compounds are not incorporated into many rock minerals, most of the nitrogen remains in the atmosphere once emitted during volcanic activity.
Oxygen (20.9%) gas is in the form of two bonded oxygens, but these bonds are not as strong as nitrogen’s. Oxygen is highly reactive, so it is taken up in the water, other atmospheric gases (carbon dioxide for example), living organisms, and many rock minerals. Because it is so reactive, it can be removed from the atmosphere for long periods of time; however, the vast amount of photosynthesizing plants provide a steady supply of atmospheric oxygen.
Argon (0.9%) is a noble or inert gas and is predominantly a radioactive decay product of potassium-40, a radioactive isotope of potassium. Minimal in Earth’s early atmosphere, argon has accumulated as potassium-40 has decayed over time.
Water vapor (0-3%) concentration is highly variable in the atmosphere, but it plays critical roles in energy flows, matter cycles, and life webs on Earth. Since hydrogen and oxygen are plentiful in the universe, water is also plentiful as it forms readily in the cold conditions of space.
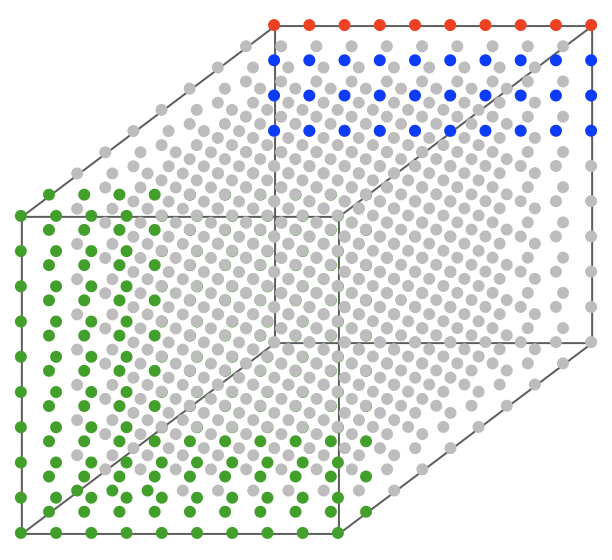
Air with 76% nitrogen (light gray), 20% oxygen (green), 1% argon (red), and 3% water vapor (blue). Note that the gases are not randomly mixed, as they are in our atmosphere.
0 Comments